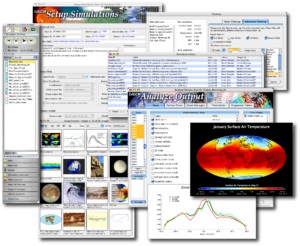
By Drew Bush and Renee Sieber
On June 10th, 2017, the New York Times reported on Gwen Beatty, a high school junior in Wellston, Ohio, who disagreed with her teacher on climate change. When he presented evidence that human emissions of greenhouse gases were causing the Earth to warm, she repeated a refrain common to many who dispute the scientific evidence tying human actions to climatic changes.
It could be natural causes. Scientists often get it wrong. Predictions of future warming could be inaccurate.
The reporter writes that if Beatty had told her math teacher that one plus one does not equal two then she would be wrong. Not all of the sciences work with the absolutes of mathematics, yet each has a way of establishing accuracy. In the public discourse on climate change—both in schools and during governmental processes—inaccuracies about climate science and global change are commonplace. A lack of public Earth and climate science understanding results in a political debate that often ignores scientific evidence of possible severe long-term impacts.
The result has been increasing concentrations of atmospheric CO2 and serious impacts for human health, the environment and the United States economy.[1] To foster a citizenry capable of engaging productively with policies addressing climate change, we believe that the public requires better understanding of the issue, how it connects to people’s lives, and what policies might benefit them in relation to it.[2],[3]
Over the past five years in McGill University’s Department of Geography and School of Environment, we’ve investigated how lessons learned through decades of science education research can be applied to improving public understandings of climate science and related mitigation/adaptation policies. Our goal has been to educate the scientific community on how to communicate climate change and to help teachers accurately present the science in school classrooms.
We reviewed the literature to look for trends and recommendations. We found that educational reformers advocate teaching climate science using the actual methods of climate scientists. This includes asking students to pose research questions, evaluate evidence-based answers or explanations, and communicate their own findings.[4], [5], [6], [7], [8], [9]
Unlike physics or chemistry, it is not easy to teach climate change this way. Climate change consists of abstract processes occurring at global and local scales. It’s difficult for students to tangibly experience climate change. To compound the problem, the causes and risks of climate change are often represented in politics and public discourse in conflicting manners. Sometimes even graduate students have trouble understanding them.[10]
Because climate scientists rely on complex procedures and technologies, new approaches to teaching climate change often adopt climate education technologies. Our approach involved an interdisciplinary group of researchers located at NASA’s Goddard Institute for Space Studies in New York, NY and McGill University’s Faculty of Education. Over the past three years, Dr. Bush has collaborated with college and secondary school educators at John Abbott College and McGill University in Montreal, QC and the American Museum of Natural History in New York, NY. He’s taught students how to conduct research on climate change using a variety of modeling technologies.
At John Abbot College, we had a control group of students and a treatment group. In our control, students learned about GCMs through a traditional lecture and worked with climate education technologies suggested by the American Association of Geographers. These included the University Corporation for Atmospheric Research’s Very, Very Simple Climate Model, NASA GISS’s Surface Temperature Analysis Page and data/visualizations from sites like the National Snow and Ice Data Center.
The treatment group of 39 students worked with Columbia University-NASA GISS’s Educational Global Climate Model (EdGCM). This software is based on an actual GCM. Dr. James Hansen first wrote about GISS’s GCM in 1983 when he used it to conduct long-range climate experiments.[11] EdGCM itself consists of a suite of user interfaces that allows students to design experiments by manipulating inputs, and then run the model and post-process and visualize more than 80 different variables. Other graduate students in Dr. Sieber’s lab have designed new interfaces for GCMs that work online and possess more intuitive user interactions.
All of the student groups posed realistic climate research questions. But only those in the treatment group interrogated the spatial components of climate impacts and its diverse relationships between human actions today and potential regional/global conditions in the future. Overall, these students demonstrated significantly greater learning gains on pre to post diagnostic exams than those in the control.
The control students showed us the power of a well-organized and clear lecture. On the post exam, they out-scored treatment students on five multiple-choice questions that tested recall of facts about GCMs. Yet only those students who had worked with EdGCM appeared highly motivated in their work and demonstrated critical thinking about the work of climate scientists and the issue of climate change.
In the New York Time story, Gwen Beatty’s father had once been a coal miner. If we are to mitigate climate change or adapt to its worst impacts, we’ll need his daughter. Her generation will require well-trained scientists, entrepreneurs, engineers and visionaries who can reshape the world’s use of energy and adapt to any social, ecological and climatic changes.
Achieving this goal means we must treat our students as individuals who possess ideas shaped by their peers and parents. To engage them with science, most will require an introduction to the scientific habits of mind common to all scientists. Such skills will be needed to navigate a world increasingly altered by changes to the Earth’s climate.
This work was supported through a Richard H. Tomlinson Fellowship in University Science Teaching and the first author’s work instructing graduate teaching workshops as a Tomlinson Project in University-Level Science Teaching Fellow at McGill University.
[1] See IPCC (2014). Summary for policymakers. In , C.B. Field, V.R. Barros, D.J. Dokken, K.J. Mach, M.D. Mastrandrea, T.E. Bilir, M. Chatterjee, K.L. Ebi, Y.O. Estrada, R.C. Genova, B. Girma, E.S. Kissel, A.N. Levy, S. MacCracken, P.R. Mastrandrea, & L.L. White (Eds.) Climate change 2014: Impacts, adaptation, and vulnerability. Part A: Global and Sectoral Aspects. Contribution of Working Group II to the Fifth Assessment Report of the Intergovernmental Panel on Climate Change (pp. 1-32) Cambridge, UK and New York, NY: Cambridge University Press.
[2] See Bord, R. J., O’Connor, R. E., & Fisher, A. (2000). In what sense does the public need to understand global climate change?. Public Understanding of Science, 9(3), 205-218.
[3] See Bord, R. J., O’Connor, R. E., & Fisher, A. (2000). In what sense does the public need to understand global climate change?. Public Understanding of Science, 9(3), 205-218.
[4] See National Research Council (NRC). (1996). National science education standards. Washington, DC: National Academies Press.
[5] See Ministry of Education [Taiwan] (2001). Standards for nine-year continuous curriculum at elementary and junior high level in Taiwan. Taipei: Ministry of Education, R.O.C.
[6] See American Association for the Advancement of Science (AAAS) (1990). Science for all Americans: Project 2061. New York, NY: Oxford University Press.
[7] See Department for Education/Welsh Office (DFE/WO) (1995). Science in the national curriculum (1995). London: HMSO.
[8] See NGSS Lead States. (2013). Next Generation Science Standards: For states, by states. Washington, DC: The National Academies Press.
[9] See Olson, S. & Loucks-Horsley, S. (Eds.) (2000). Inquiry and the national science education standards: A guide for teaching and learning. Washington, DC: National Academies Press.
[10] See Sterman, J. D. (2008). Risk communication on climate: Mental models and mass balance. Science, 322(5901), 532-533.
[11] See Hansen, J., Russell, G., Rind, D., Stone, P., Lacis, A., Lebedeff, S., Ruedy R. & Travis, L. (1983). Efficient three-dimensional global models for climate studies: Models I and II. Monthly Weather Review, 111(4), 609-662.